Einstein's Miracles, Part 2: Atoms
- Caleb Todd
- Apr 18, 2022
- 5 min read
By Caleb Todd
Atoms are a deeply familiar part of our natural world. Their name is taken from the Greek atomos (meaning ‘indivisible’, despite the fact that atoms have constituent pieces into which they can be divided) because they constitute the fundamental unit of a chemical element. If you take a helium atom and try to break it up — divide it — what you have left is no longer helium.
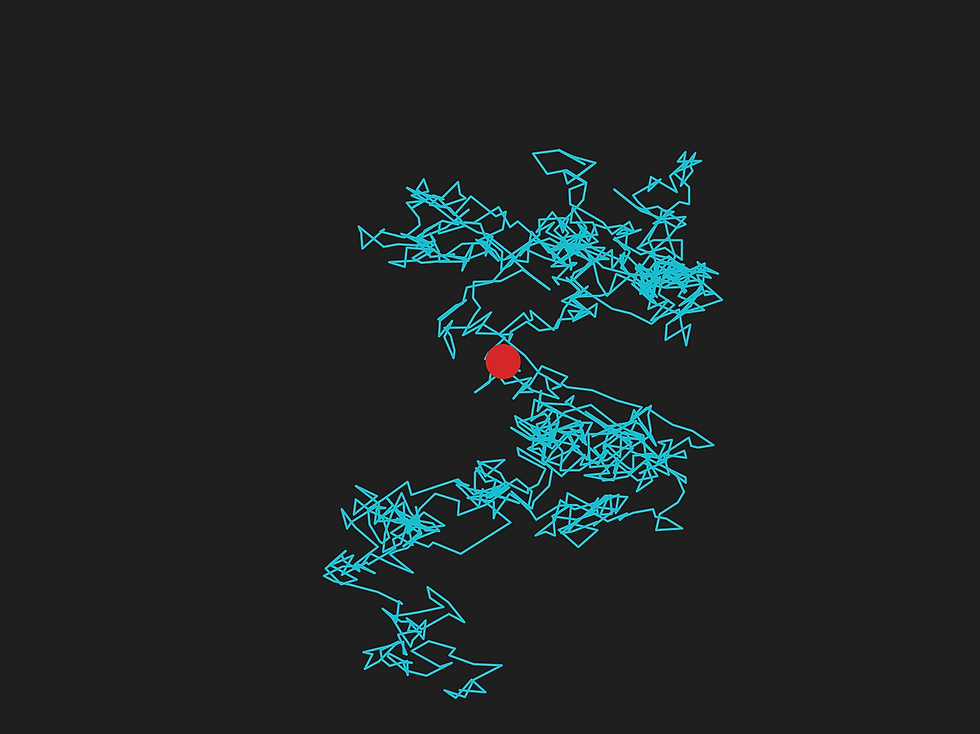
Figure 1: A simulation of a particle undergoing Brownian motion. The red particle jittered randomly along the blue curve.
We are quite comfortable, these days, with the idea that atoms are matter’s building blocks, but universal acceptance thereof is actually a relatively recent development. While the idea of atoms goes back to ancient Greece, where scholars like Leucippus and Democritus proposed indivisible units of substance, these were philosophical arguments, not scientific [1]. Those who were more rigorous had to wait until around 1800 AD before atomic theory really developed as a science [1], and when our frizzy-haired protagonist came along in the early 20th century, there was still debate over its validity.
In our last issue¹, we began the story of Einstein’s annus mirabilis papers by highlighting his work on the quantum nature of light. He helped launch the quantum revolution which subsequently redefined and recontextualised all of physics. The significance of that paper was only recognised slowly, though, so Einstein decided that if one revolutionary paper per year wasn’t enough, he’d just have to write two². As such, he turned his mind to the matter of matter and published ‘Über die von der molekularkinetischen Theorie der Wärme geforderte Bewegung von in ruhenden Flüssigkeiten suspendierten Teilchen’ (‘On the movement of small particles suspended in a stationary liquid demanded by the molecular-kinetic theory of heat’) in Annalen der Physik, 18 July 1905 [2].
At the heart of Einstein’s discussion lies the phenomenon of Brownian motion³. If you suspend a very light particle (like a mote of dust, for example) in a fluid and place it under a microscope, you will see it randomly zigzag and jitter [3], like in Fig. 1. Though no objects are visibly colliding with it or exerting a force on it, the particle is still continuously changing its motion. Is this a violation of Newton’s first law of motion? Certainly not. Instead, we might suspect that there are invisible objects colliding with the particle causing its variation in speed and position — and if they are invisible, they must be very small indeed. Perhaps Brownian motion is caused by atoms, which were proposed by the chemist John Dalton to explain how some substances can combine to make other substances. This is the explanation that Einstein proposed, but proposals and proofs are two very different beasts.
At the same time, there was another thread in physics running parallel to the question of atoms: what is heat? What property of a substance makes it hot or cold? For a long time, scientists thought that there was an invisible fluid that imbued heat to the objects around which it flowed⁴. That notion was dismissed, however, when James Joule demonstrated that heat was just another form of energy⁵ [4] — but what kind of energy? This is where the ‘molecular-kinetic theory of heat’ in Einstein’s title comes in. In this theory, heat energy is really kinetic energy; that is, the energy associated with motion. In particular, it purports that heat is the kinetic energy of the atoms (or molecules) that make up a substance. You can now, perhaps, see how these threads tie together. If atoms exist and the kinetic theory of heat is correct, Brownian motion can be directly explained as collisions between the jittering particle and hot atoms in motion⁶.
It seems a very cogent theory, but that in and of itself does not place these questions beyond doubt. We need something measurable that could experimentally validate Einstein’s conclusions. For this reason, one of the most significant parts of his paper is a mathematical expression for how quickly particles undergoing Brownian motion spread out from their initial positions. As it turns out, this average speed depends on the fundamental properties of the atoms being theorised about. So, by measuring Brownian motion, a physicist could help substantiate (or discredit) the kinetic theory of heat.
Einstein did not have available to him sufficient data to actually draw a conclusion. Rather than trying to do the experiment himself, he simply concluded his paper by saying (in German), “Let us hope that a researcher will soon succeed in solving the problem posed here, which is of such importance in the theory of heat!” [2]. Fortunately for Einstein (and all other theoretical physicists), there are plenty of experimentalists who are willing to actually check whether the nonsense they write down is true. In this case, it was a Frenchman by the name of Jean Baptiste Perrin, whose experiments concluded (lo and behold) that Einstein’s predictions were correct⁷ [5].Atoms do exist, the molecular-kinetic theory of heat works, and we’ve never looked back since.
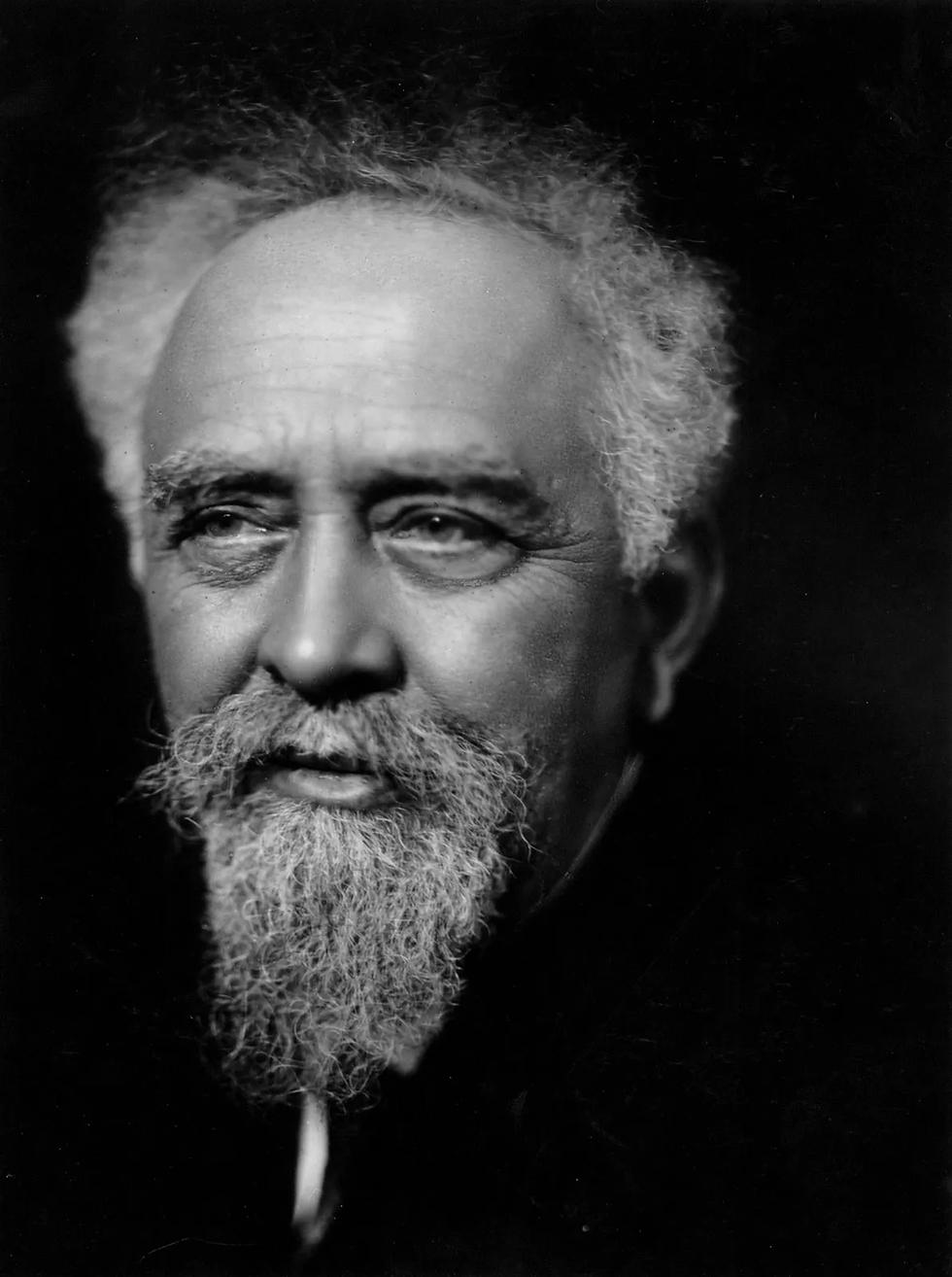
Figure 2: Jean Baptiste Perrin, French physicist and winner of the 1926 Nobel Prize for demonstrating the existence of atoms. Image taken from Encyclopædia Britannica.
Though it would be disingenuous to suggest that this result was totally surprising to the physics community — atoms and kinetic heat were well-regarded theories — it was absolutely still a controversial topic when Einstein’s paper was submitted. The importance of atomic theory need hardly be restated, and Jean Baptiste Perrin was awarded a Nobel Prize for his experimental verification of Einstein’s theory [5]. Note that this is the second of Einstein’s 1905 papers connected to a Nobel prize (although not for Albert himself, this time). Einstein is two-for-two⁸. This paper on Brownian motion is often overlooked in the annus mirabilis because of how revolutionary quantum theory and special relativity (the subjects of the other three 1905 papers) are, but that is somewhat unfair to an incredibly significant paper. We are now living in the ‘Atomic Age’, but barely a century ago we weren’t even sure that atoms existed.
For the second time in just two months, Albert Einstein had changed the way we saw the world — but he wasn’t finished yet. In the next edition of the UoA Scientific, we will watch Einstein quite literally challenge the structure of reality itself.
¹ Available on our website.
² I might be projecting motivations a
little bit here.
³ Thankfully this has nothing to do with digestion. It is named after its discoverer Robert Brown
⁴ Physicists often invent imaginary fluids to grapple with phenomena they don’t understand, as we will see in the next part of this series when we discuss the aether and light speed.
⁵ Essentially all engines and electricity generators depend on this principle. The SI unit of energy is named the Joule in his honour.
⁶ The hotter the atoms, the more jittering they cause. Much like in night clubs (or so I am told).
⁷ You’re shocked, I’m sure.
⁸ Although the prizes themselves were, of course, awarded far later than 1905.
References
S.B. McGrayne, J. Trefil, and G.F. Bertsch, “atom,” 2022. [Online]. Available: https://www.britannica.com/science/atom. [Accessed: 25- March- 2022].
A. Einstein, “Uber die von der molekularkinetischen theorie der warme geforderte bewegung von in ruhenden flussigkeiten suspendierten teilchen,” Annalen der Physik, vol. 322, no. 8, pp. 549–560, 1905.
R. Feynman, "The Brownian Movement," The Feynman Lectures of Physics, vol. I, pp. 41–1, 1964.
J.P. Joule, “On the Mechanical Equivalent of Heat”, in Report of the British Association for the Advancement of Science, 15th Meeting, 1845, pp. 536.
M. Dardo, Nobel Laureates and Twentieth-Century Physics, Cambridge: Cambridge University Press, 2004, pp. 114–116
Comments