Explained: The Rise and Fall of Gymnosperms
- Nina de Jong
- Aug 17, 2021
- 7 min read
Updated: Sep 27, 2021
By Nina de Jong
Gymnosperms are a division of plants that have a long and proud history of worldwide distribution and evolution. Historically, gymnosperms dominated the earth’s flora during the early and mid-Mesozoic era, around 250-150 million years ago. However, now there are only about 1,000 species of gymnosperms – this includes 5 orders of plants: the funky cycads (Cycadales), the strange Gnetales, the lonely ginkgo (Ginkgoales) and all of the conifers (Pinales and Cupressales). This history of being once widespread and dominant, and now being a much smaller species pool, indicates a fascinating evolutionary and ecological history for gymnosperms.
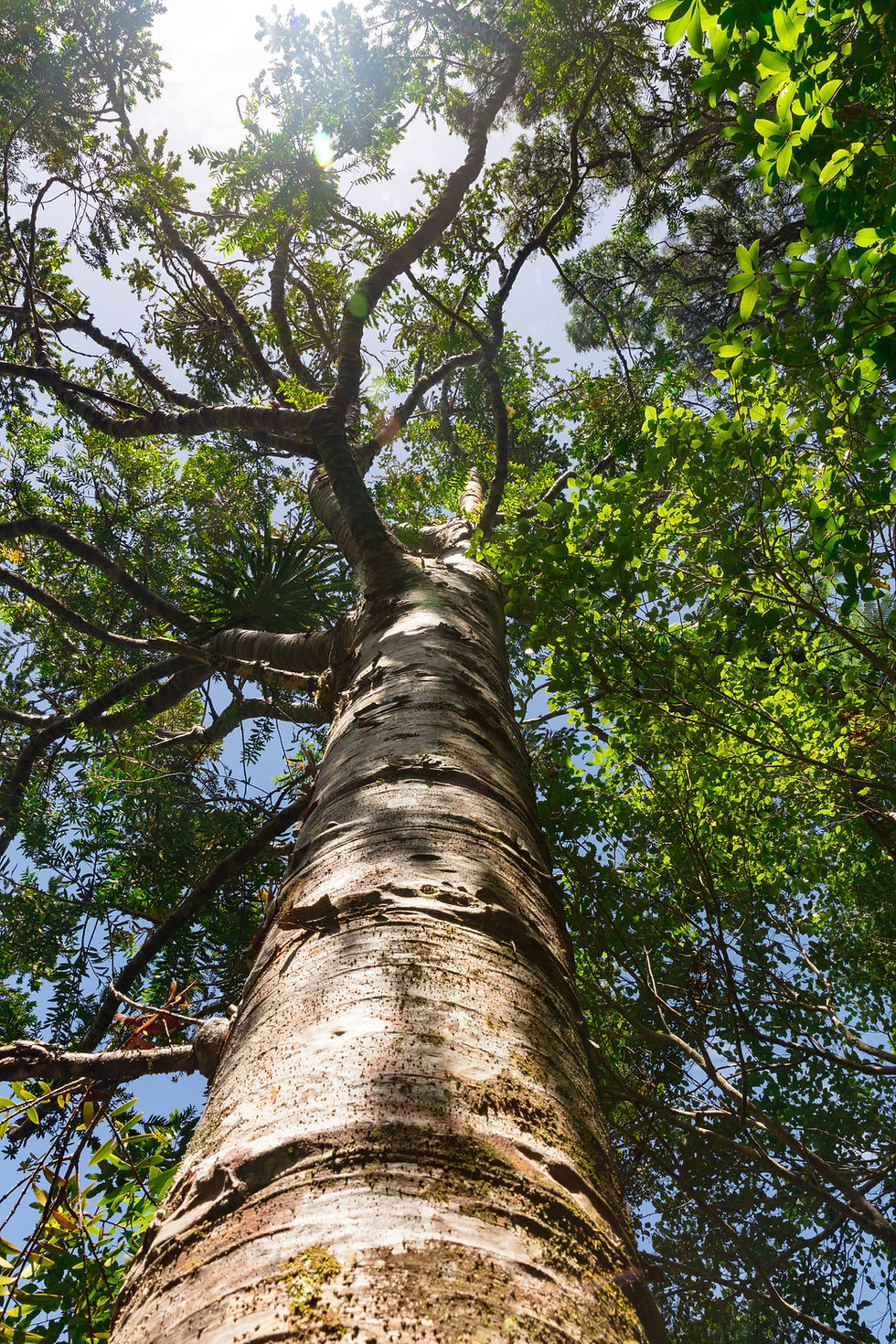
Agathis australis is a beautiful example of the magnificence of gymnosperms. Photo by Jon Moore on Unsplash.
Gymnosperms were some of the first plants to develop wood for mechanical support. Although wood had appeared before the gymnosperm lineage, ancient forms of wood served primarily to help plants with water conduction [1]. Gymnosperms were the first plants for which wood did not just provide efficient water conducting tissue (xylem), but also structural support. The gymnosperm lineage was, therefore, able to grow taller trees with larger canopies and has given rise to the first trees as we know them today [2].
Gymnosperms represent the evolution of the first seed plants. Before the development of the seed, plants had a very complicated reproductive routine, involving alternating generations of sporophytes (diploid individuals, with both sets of chromosomes) and gametophytes (haploid individuals, with only one set of chromosomes), where gametophytes release eggs and sperm that need to find each other through water, to produce diploid individuals (sporophytes). Plants such as ferns and mosses still go through this process, but gymnosperm seeds have compressed the gametophyte to microscopic size. This has allowed the haploid stage of the life cycle to be done in a safe environment while still attached to the parent plant, and with no need for water. The seeds can then be easily dispersed by various methods, including via wind and animals. This has meant that gymnosperms no longer have to grow in environments that have an abundance of water for reproduction, and many of today’s gymnosperms can survive in very harsh, extreme environments. Gymnosperms first evolved during the Carboniferous period, during which Pteridophytes (ferns and fernlike plants) were the dominant group of plants [3]. A major mass extinction event at the end of the Paleozoic (250 mya) meant that gymnosperms, with their new developments for growth and reproduction, were well placed to have a prominent position in the composition of plant communities worldwide during the Mesozoic era [4]. The first ancestors of today’s gymnosperms evolved about 311-212 mya, which is a long time ago when compared to the evolution of the other seed plants, the angiosperms, which evolved 125-100 mya [5]. Gymnosperms, despite undergoing some speciation and niche-shifting in response to environmental change, have a well conserved evolution and are quite similar to their ancestors [6]. This is especially the case for gymnosperms of the southern hemisphere, where the warmer and wetter conditions haven’t forced such extreme selective pressures on species [7]. As a result, gymnosperms remained dominant throughout the Mesozoic for about 100 million years.
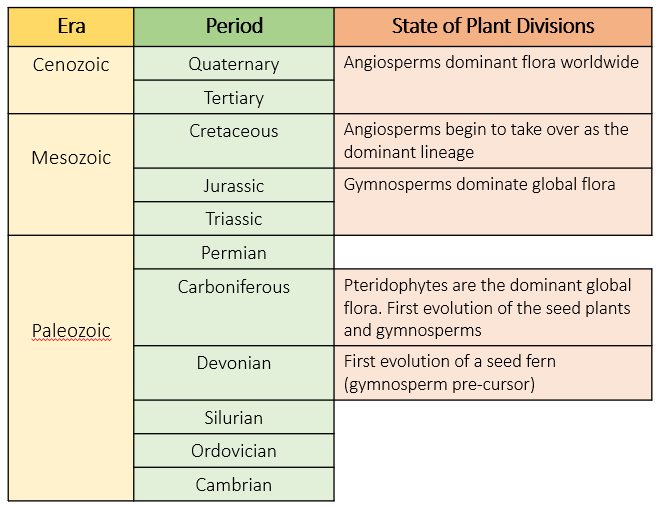
The stages of gymnosperm evolution through the last 350 million years.
However, gymnosperm dominance was not to last, and along with decreasing atmospheric CO2 , the rise of a new lineage of plants is largely attributed to the decline of gymnosperms [8], and it is impossible to describe the history of gymnosperms without also talking about angiosperms. Today, the world is dominated by the angiosperms, a group of seed plants that far outstrip the gymnosperms in terms of modern species diversity, with over 300,000 species compared to the 1000 species of gymnosperms. The angiosperms are thought to have evolved from an extinct lineage of gymnosperms in the Cretaceous, and their evolution is characterised by a huge and abrupt diversification of species to occupy new ecological niches in the Late Cretaceous and Early Tertiary [8,5]. These new species managed to outcompete gymnosperms and supersede their dominance by the Tertiary period. But gymnosperms had been the dominant plant group for 100 million years, thriving across the world. So how did this abrupt and convincing switch of dominance happen?
Angiosperms underwent an evolutionary radiation in the Late Cretaceous, 100 million years ago. In many ways, gymnosperms walked so that angiosperms could run. The developments gymnosperms had made, such as tracheid xylem and seeds, were the building blocks for angiosperms to develop even more efficient and creative methods of growth and reproduction.
The gymnosperm cones and seeds are the evolutionary precursor to flowers and fruits, which are many people’s and animals’ favourite thing about plants. Angiosperm flowers and fruits are thought to be the drivers of much of the diversity among angiosperms [9]. Flowers and fruits are highly susceptible to selective pressures, as flowers serve as sites for pollination, which can be carried out by animals, wind, and other forces [10]. Animals, in particular, apply specific and strong selective pressures that promote diversification of flower shape and colour, and this allows angiosperms to diversify under co-evolution [9,11]. Flowers are also thought to allow angiosperms to persist in smaller populations, as pollination is more targeted and so the plants do not have to be as abundant to ensure pollination occurs [9,11].
Atmospheric CO2 declined during the Cretaceous, and this trend selected for plants that left their stomata open longer, to receive enough CO2 for photosynthesis. When stomata are open longer, plants need more water to make up for the water lost in transpiration. This led to selective pressure for more efficient water conductance [12,13]. While gymnosperm xylem, known as tracheids, function as both structural and conductive tissue, angiosperms differentiate these tissues into vessel elements and supportive fibres. This enables the specialisation of vessel elements for more efficient water conduction [14]. In turn, increased water availability allowed angiosperms to develop broader leaves that have a greater photosynthetic capacity [3]. Although podocarps with flattened leaves can compete with angiosperms in some tropical understoreys [15], today gymnosperms are largely out-competed by angiosperms in lowland tropical rainforests, habitats which select for plants with large leaves that have a high photosynthetic capacity [15].
With these adaptations, angiosperms were able to rapidly spread and dominate the world’s flora. And yet, the story of the gymnosperms is not over! Gymnosperms are still around today. If angiosperms were so unassailably dominant, there would be no extant gymnosperms. Somehow, this group continued to thrive successfully in the face of their seemingly unstoppable cousins.
Mostly, gymnosperms thrive in the landscape by differentiating away from direct competition against angiosperms and their greater photosynthetic capacity [15]. For example, in Aotearoa, the coexistence of conifers and angiosperms can be attributed to a regeneration differentiation along a shade-tolerant versus stress-resistant niche differentiation [16]. Here, angiosperms outcompete conifers in both shady and light environments due to a higher photosynthetic capacity and growth rate, and so conifers occupy more stressful, exposed regeneration sites while angiosperms occupy more sheltered sites in the forest interior [16]. In the northern hemisphere, gymnosperm species are dominant in ecosystems in extreme conditions – in very cold climates such as boreal forests and coniferous forests in central continents.
Gymnosperms are a fascinating and ancient group, and their history shows how groups can expand and contract in dominance and diversity as conditions and biotic competition changes. With the onset of climate change, warmer conditions at the poles and more unpredictable weather, what does the future hold for gymnosperms?
References
[1] Strullu-Derrien, C., Kenrick, P., Tafforeau, P., Cochard, H., Bonnemain, J. L., Le Hérissé, A., ... and Badel, E. (2014). The earliest wood and its hydraulic properties documented in c. 407-million-year-old fossils using synchrotron microtomography. Botanical Journal of the Linnean Society, 175(3), 423-437.
[2] Wilson, J. P., & Knoll, A. H. (2010). A physiologically explicit morphospace for tracheid-based water transport in modern and extinct seed plants. Paleobiology, 36(2), 335-355.
[3] Wilson, J. P. (2013). Modeling 400 million years of plant hydraulics. The Paleontological Society Papers, 19, 175-194.
[4] DiMichele, W. A., Hook, R. W., Beerbower, R., Boy, J. A., Gastaldo, R. A., Hotton III, N., ... & Sues, H. D. (1992). Paleozoic terrestrial ecosystems. Terrestrial ecosystems through time. University of Chicago Press, Chicago, 205-325.
[5] Berendse, F., & Scheffer, M. (2009). The angiosperm radiation revisited, an ecological explanation for Darwin’s ‘abominable mystery’. Ecology Letters, 12(9), 865-872.
[6] Wang, X. Q., & Ran, J. H. (2014). Evolution and biogeography of gymnosperms. Molecular phylogenetics and evolution, 75, 24-40.
[7] Leslie, A. B., Beaulieu, J. M., Rai, H. S., Crane, P. R., Donoghue, M. J., & Mathews, S. (2012). Hemisphere-scale differences in conifer evolutionary dynamics. Proceedings of the National Academy of Sciences, 109(40), 16217-16221.
[8] Condamine, F. L., Silvestro, D., Koppelhus, E. B., & Antonelli, A. (2020). The rise of angiosperms pushed conifers to decline during global cooling. Proceedings of the National Academy of Sciences, 117(46), 28867-28875.
[9] Lunau, K. (2004). Adaptive radiation and coevolution— pollination biology case studies. Organisms Diversity & Evolution, 4(3), 207-224.
[10] Sauquet, H., & Magallón, S. (2018). Key questions and challenges in angiosperm macroevolution. New Phytologist, 219(4), 1170-1187.
[11] Specht, C. D., & Bartlett, M. E. (2009). Flower evolution: the origin and subsequent diversification of the angiosperm flower. Annu. Rev. Ecol. Evol. Syst., 40, 217-243.
[12] Lusk, C. H., Wright, I., and Reich, P. B. (2003). Photosynthetic differences contribute to competitive advantage of evergreen angiosperm trees over evergreen conifers in productive habitats. New Phytologist, 160(2), 329-336.
[13] Brodribb, T. J., Feild, T. S., and Jordan, G. J. (2007). Leaf maximum photosynthetic rate and venation are linked by hydraulics. Plant physiology, 144(4), 1890-1898.
[14] Sperry, J. S., Hacke, U. G., and Pittermann, J. (2006). Size and function in conifer tracheids and angiosperm vessels. American journal of botany, 93(10), 1490-1500.
[15] Brodribb, T. J., Pittermann, J., and Coomes, D. A. (2012). Elegance versus speed: examining the competition between conifer and angiosperm trees. International Journal of Plant Sciences, 173(6), 673-694.
[16] Lusk, C. H., Jorgensen, M. A., and Bellingham, P. J. (2015). A conifer–angiosperm divergence in the growth vs. shade tolerance trade-off underlies the dynamics of a New Zealand warm-temperate rain forest. Journal of Ecology, 103(2), 479- 488.
Yorumlar