The Algol Paradox: How Do Stars Age?
- Aimee Lew
- Apr 18, 2022
- 6 min read
By Aimee Lew
Algol is perhaps one of the most storied stars in Earth’s night sky.
Going by Beta Persei or the Demon Star (from Arabic: ra's al-ghūl, just like the DC villain), for thousands of years, Algol was considered an omen of death and destruction [1]. The reason for this may have been its variability, or the periodic fluctuation of its brightness. In astronomy, the apparent magnitude is a measure of a star’s brightness to earthbound observers. Algol’s apparent magnitude dips approximately every three days, which — if perhaps one squints and drinks — could look like the slowly blinking eye of a harbinger of death.
With the advent of telescopy and spectroscopy, astronomers learned in the 1880s that Algol was not one star, but multiple [2, 3]. It comprises three bodies that blur into a bright dot before us. Two of Algol’s components, β Persei A and B, orbit and eclipse each other. The passage of one body in front of the other, with respect to the line to Earth, decreases the apparent magnitude.
With more technological breakthroughs in the twentieth century, , the catalogue of star masses, distances from Eart
h, temperatures, compositions and age grew and grew. Astrophysicists attempted to put together a description for the evolution of stellar bodies. The theory went — and still goes, with more nuanced addendums — that more massive stars age quicker. But the Algol binary was an anomaly: the smaller body had advanced, and the larger body remained in an earlier stage of stellar evolution. Thus, the Algol Paradox was born [4].
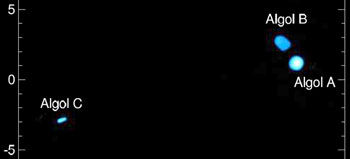
Figure 1: The Algol system on 12 August 2009. Image from the CHARA (Center for High Angular Resolution Astronomy) array in California.
To understand why β Persei A and B supposedly defied the trends of stellar evolution, we first need to understand stellar evolution. Think of a star like a furnace. From nebulae, they are born with a certain amount of ‘fuel’ (hydrogen) in their cores. The amount of fuel a star gets is determined by its mass. In their cores, nuclear reactions are taking place at extraordinary rates and scales. The light of a star comes from the energy output of converting protons into helium nuclei. More massive stars burn fuel at larger rates and scales, so they are hotter, brighter, and deplete their hydrogen sources more quickly [5].
While a star remains in the fuel-burning early stage of their lifetime, they sit on the main sequence. The main sequence is a diagonal band of stars on the Hertzsprung-Russell (HR) diagram [5].

Figure 2: The Hertzsprung-Russell diagram with data from the Hipparcos and Gliese catalogues. HR diagrams compare the luminosities, or brightness, of a star to its stellar colour, or effective temperature. In the upper-left are the hot and bright stars, which appear as white-blue, and in the lower-right are the (relatively) cool and dim stars, which appear as orange-red. Underneath the main sequence are the white dwarves—extremely dense stars that are in their final evolutionary state—and above are the red giants and supergiants.
Astronomers used to think that the main sequence showed the pathway of stellar evolution. Stars would begin their lives in the upper-left, hot and bright, and cool over time, falling into the lower-right corner [5]. But the main sequence of the HR diagram is no more an evolutionary pathway than the band of the Milky Way; both are simply smatterings of stars at some given point in time. All stars are born on the main sequence, their position dictated by brightness and temperature, which is in turn dictated by mass. For as long as nuclear fusion persists in their cores, which is about 90% of their lifetime, they don’t move significantly along the diagonal [6]. Larger stars inhabit the upper-left and leave the main sequence fast (cosmically speaking) after a few million years. Smaller stars inhabit the lower-right and can burn for billions of years, like our Sun.
When stars deplete their sources of hydrogen, they evolve. Lower mass stars expand into red giants (large, less dense and cool), then shrink into white dwarves (small, dense and hot) which will eventually radiate away all of its energy and wink out. Higher mass stars expand into red supergiants (very large and very cool) that will expand until the outward radiation pressure drops lower than the inward force of gravity. At that moment, red supergiants will crumple and supernova, creating atomic nuclei anew.
In the Algol system, astrophysicists expected the two orbiting bodies to evolve as two individuals, but the observations seemed to suggest the opposite was true [7]. There is a smaller star in the giant phase and a larger star still on the main sequence. To understand the contradiction of Algol A and B’s behaviour, we need to focus on two things: red giants and gravity.
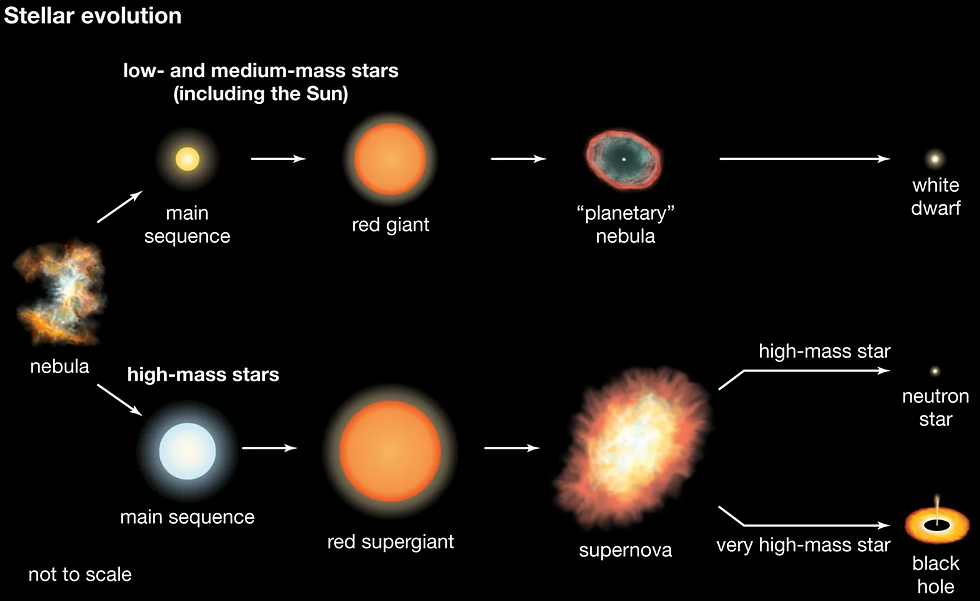
Figure 3: Stellar evolution diagram. Image from Encyclopaedia Britannica, 2012.
Notice that whether a star begins with more mass or less, both classifications have a period of expansion into either a subgiant, giant or supergiant star, which is a later but not final stage of stellar evolution. In binary star systems, the Roche lobe helps to shed light on the Algol Paradox. The Roche lobe defines the region around each star in which matter is gravitationally bound to that star. Roughly teardrop-shaped, the intersection of each lobe is called the Lagrange point. In Algol-type binaries, it’s possible for expanding red giants to fill their Roche lobe [8]. When that happens, matter can be transferred away from the initial stellar body. Roche lobe overflow (RLOF) is the culprit for this anomalous behaviour [9].
Astrophysicists now know that binary star systems like Algol A (a hot blue-white main sequence star) and B (a cooler orange subgiant) begin as two close-range stars of the same age and composition—having formed from the same nebula—with different masses. The larger star ceases nuclear fusion and starts expanding into a red giant earlier than the smaller star, as suggested by conventional stellar evolution. At this point, orbiting material that fills the larger star’s Roche lobe and surpasses the Lagrange point falls into the gravitational well of the smaller star [10]. As stellar expansion continues, more mass transfers to the smaller body until there appears to be a less massive star (Algol B at roughly 0.7 solar masses) that has burned through all its hydrogen and a more massive star (Algol A at roughly 3.2 solar masses) that is still trucking along [11].
Solved in the twentieth century, the Algol Paradox helped to shed light on the behaviour and evolution of binary star systems. Algol-type binaries remain a hotbed of academic interest, classified as cases “where the less massive donor fills its Roche lobe, the more massive gainer does not fill its Roche lobe and is still on the main sequence and the donor is the cooler, fainter and larger star” [9]. Meanwhile, training a closer and closer eye on Algol revealed in 2020 that the system might contain more bodies than anyone, thousands of years ago, staring up at the blinking Demon Star, could have thought [12]. The history of Algol unfolds alongside the discipline of astronomy and astrophysics, marking with pinpricks of light what we thought we knew, what we do know, and what we have yet to discover.
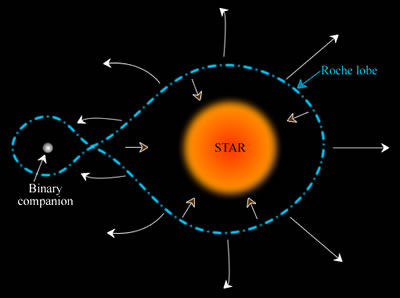
Figure 4: Roche lobes of a binary star system. Image from COSMOS, the SAO Encyclopaedia of Astronomy, Swinburne University.
References
S. R. Wilk, "Mythological evidence for ancient observations of variable stars", The Journal of the American Association of Variable Star Observers, vol. 24, no. 2, pp. 129-133, 1996. [Accessed 21 March 2022].
[E. Pickering, "Dimensions of the Fixed Stars, with Especial Reference to Binaries and Variables of the Algol Type", Proceedings of the American Academy of Arts and Sciences, vol. 16, p. 1, 1880. Available: 10.2307/25138595.
A. Batten, "Two Centuries of Study of Algol Systems", International Astronomical Union Colloquium, vol. 107, pp. 1-8, 1989. Available: 10.1017/s0252921100087625.
I. Pustylnik, "The early history of resolving the Algol paradox", Astronomical & Astrophysical Transactions, vol. 15, no. 1-4, pp. 357-362, 1998. Available: 10.1080/10556799808201791.
K. Lang, The life and death of stars. Cambridge [England]: Cambridge University Press, 2013.
"How do scientists determine the ages of stars?", Scientific American, 1999. [Online]. Available: https://www.scientificamerican.com/article/how-do-scientists-determi/. [Accessed: 21- Mar- 2022].
I. Pustylnik, "Resolving the Algol Paradox and Kopal’s Classification of Close Binaries with Evolutionary Implications", Astrophysics and Space Science, vol. 296, no. 1-4, pp. 69-78, 2005. Available: 10.1007/s10509-005-4379-1.
P. Davis, L. Siess and R. Deschamps, "Binary evolution using the theory of osculating orbits", Astronomy & Astrophysics, vol. 570, p. A25, 2014. Available: 10.1051/0004-6361/201423730.
W. Van Rensbergen, J. De Greve, N. Mennekens, K. Jansen and C. De Loore, "Mass loss out of close binaries", Astronomy and Astrophysics, vol. 510, p. A13, 2010. Available: 10.1051/0004-6361/200913272.
B. Paczynski, "Evolutionary Processes in Close Binary Systems", Annual Review of Astronomy and Astrophysics, vol. 9, no. 1, pp. 183-208, 1971. Available: https://www.annualreviews.org/doi/10.1146/annurev.aa.09.090171.001151.
F. Baron et al., "Imaging the Algol triple system in the H band with the CHARA Interferometer", The Astrophysical Journal, vol. 752, no. 1, p. 20, 2012. Available: 10.1088/0004-637x/752/1/20.
L. Jetsu, "Say Hello to Algol's New Companion Candidates", The Astrophysical Journal, vol. 920, no. 2, p. 137, 2021. Available: 10.3847/1538-4357/ac1351.
Comments