The James Webb Space Telescope Time Machine
- Celina Turner
- Feb 7, 2022
- 8 min read
Updated: Apr 18, 2022
By Celina Turner
It’s a question everyone asks sooner or later: how did the universe start? How are planets and stars and galaxies created? What else is out there? Throughout the thousands of years during which humans have had consciousness that allowed them to question the origins and vastness of the universe, they lacked an ability to find definitive answers. However, we are now living in a time where the possibility of seeing the universe at its earliest is becoming reality. In the last century, astronomy has made great strides in our understanding of what is possible, and what tools are needed to discover the creation of both the universe and the structures within it. The James Webb Space Telescope (JWST) has been a twenty-year project costing $10 billion (USD), with the aim of providing us the information that shows us the beginning of our universe [1]. But first, let’s back up a bit to understand how it will be able to do that, and why it is a technological marvel.
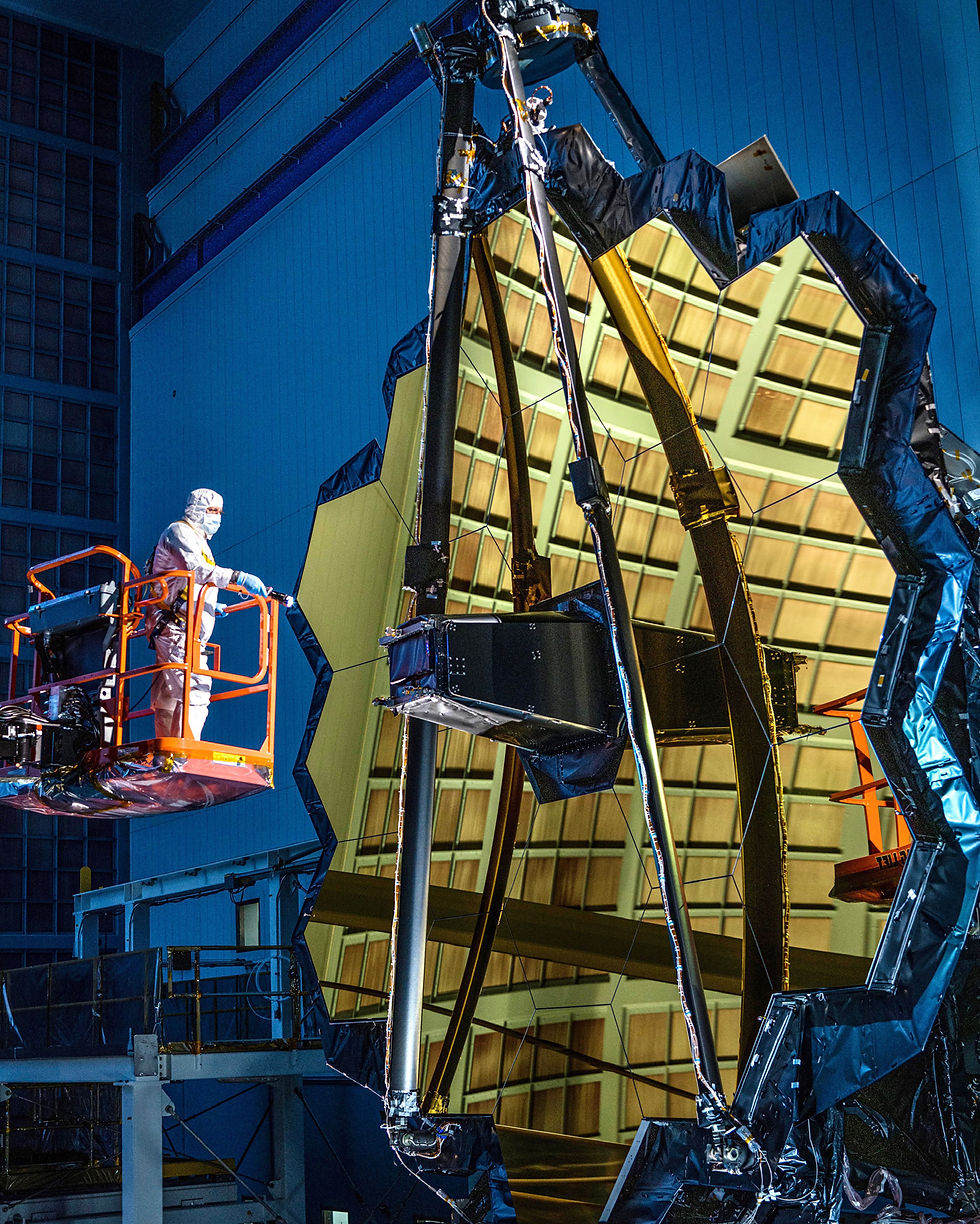
Image credit: NASA/Chris Gunn
We know there was a beginning to the universe — otherwise, as Olbers’ Paradox points out, the sky would be perpetually blindingly-bright from infinite stars in infinite directions sending infinite rays of light towards Earth. The Big Bang theory explains how our universe was created: in a fraction of a second (1/1043 of a second, to be precise), the universe exploded into existence— just a billion-degree hot pool of protons and neutrons and intense radiation that continuously expanded to fill a limitless space [2]. Particles collided to create elements such as hydrogen and helium, and eventually the first star blazed into existence.
Because light takes time to travel over distances, we can look back in time by looking at light originating from really far points; i.e. if you were to look at a star that is one light-year away, one year is required for the light from that star to be seen. Thereby, looking at stars that are billions of lightyears away shows us what they were like billions of years ago, as their light has only just reached us. However, just as the Doppler Effect changes the wavelength of an ambulance’s siren when it passes from behind you to in front of you, the expansion of the universe stretches the wavelengths of the light traveling to us— known as redshift. This puts some of the possible observations in the infrared spectrum or lower instead of the optical spectrum as observable light. Even high-energy radiation that was released in the Big Bang, which is still found to be travelling across the universe, has had its wavelengths stretched into the microwave spectrum. This leftover radiation was found (accidentally) in 1965; now known as the Cosmic Microwave Background, this discovery is what ultimately convinced those who were on the fence about the Big Bang— that the universe was not a steady state, and that some beginning had to exist.
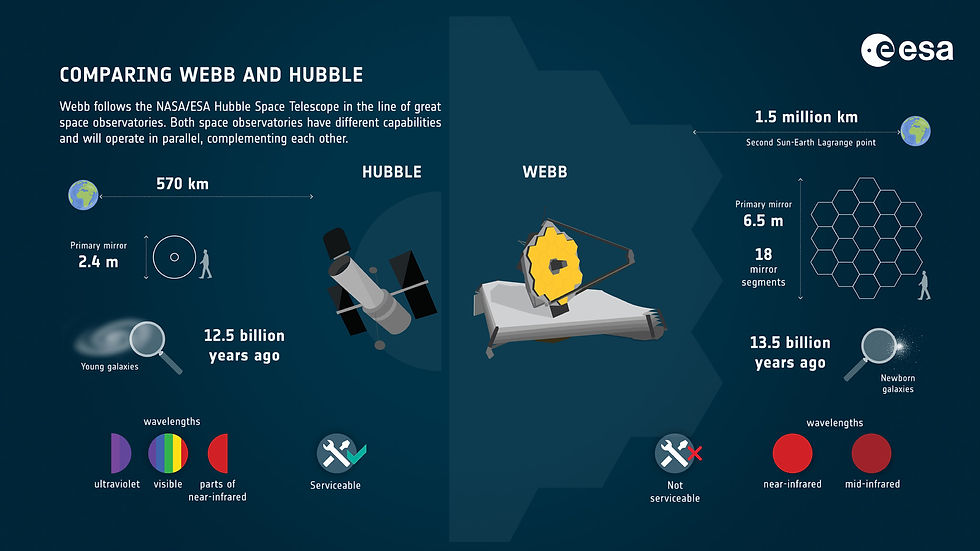
Image: ESA/NASA
The Hubble telescope gave us a much better view into deep space, providing data that allowed us to deepen our understanding of some intricacies relating to what happened after the beginning, but it simply wasn’t enough to answer questions fully. In 1995, Bob Williams (the then director of the Space Telescope Institute) used his allocated time with Hubble to do something many astronomers considered a waste at the time— he pointed it at the darkest spot in the sky to see if it was truly dark [3]. The 100 hours of exposure time would allow the telescope to soak up as much faint light that may shine from within the black void, and indeed, there was light. Now known as the Hubble Deep Field photo, his resulting image changed how astrophysicists understood the evolution of galaxies. Thousands of galaxies revealed themselves from the depths of the tiny fraction of the sky. The seemingly endless collection of worlds of different ages painted a picture of the universe over millions to billions of years. A colleague of Bob Williams stated that “what Hubble succeeded in doing with the Hubble Deep Field is finding that there were galaxies at redshifts much higher than we thought” [3]. This conclusive proof of an evolving universe, however, only led to more questions about its very beginning, and how galaxies form at all. Seeing the universe closer in time to the Big Bang would require light that not even Hubble can detect. Interestingly, the idea of a Hubble successor that could look further back in time began to form even before the launch of the Hubble itself [3]. The majority of celestial bodies emit infrared radiation, but this of course also includes Earth, which drowns out anything that equipment on the ground could detect [4]. Thus, any invention used for the purpose of seeing the cosmos in infrared would need to be off-planet, and the concepts for such a telescope were already being discussed before Hubble was in orbit. With the discoveries of the Hubble Deep Field, such as that the oldest galaxies would be redshifted so far that there would be no other way to view them, the need for the James Webb Space Telescope became clear.
Hubble uses a mirror with approximately 4.5 m2 of collecting area, and operates in the optical and ultraviolet spectrums while orbiting Earth [5]. The JWST instead will have approximately 25.4 m2 of collecting area, while operating in the infrared spectrum from the L2 Sun-Earth Lagrange point [5]. Dr Elizabeth Howell explains: “A Lagrange point is a location in space where the combined gravitational forces of two large bodies, such as the Earth and the sun or the Earth and the moon, equal the centrifugal force felt by a much smaller third body. The interaction of the forces creates a point of equilibrium where a spacecraft may be ‘parked’ to make observations” [6]. The L2 point will enable the JWST to sit in perpetual darkness with the sun being blocked by the Earth. Aside from there being no infrared radiation produced by the sun or the Earth, there are the added benefits of this placement such as allowing for constant observations and helping keep the JWST incredibly cold, which is crucial for its detectors to ensure accurate readings as they “need to be at a temperature of less than 7 kelvin to operate properly” [7]. However, this means that if reparations are needed, as Hubble has in the past, the JWST will be out of luck as it is too far away from Earth to send a service mission. Knowing that mistakes will not be able to be fixed has required every aspect to be expected to work flawlessly on its first and only try [1].

Image: ESA/NASA
The threat of having a single attempt becomes more intimidating when considering the technological requirements JWST needs to fulfill while adhering to the limitations of bringing the rocket into space. In order to have as much precision as the Hubble telescope, the mirror of the JWST needs to be much larger, as the wavelengths of the light it is capturing are larger and fainter. But with the diameter of the mirror at 6.5 m and the diameter of the Ariane 5 rocket carrying it being only 5.4 m, engineers needed to design a mirror that could fold for launch, unfold itself in space, and align each mirror with incredible precision [8]. "Aligning the primary mirror segments as though they are a single large mirror means each mirror is aligned to 1/10,000th the thickness of a human hair. What's even more amazing is that the engineers and scientists working on the Webb telescope literally had to invent how to do this," says Lee Feinberg, the Webb Optical Telescope Element Manager [9]. The solution includes 18 hexagonal mirrors, each 1.32 m wide and made of beryllium with gold plating, that can unfold similarly to a flower blossoming [9]. Additionally, the sunshield will also need to be able to unfold in space. It may be 22 m by 12 m in size (roughly the size of a tennis court), but it is made of only five thin layers of kapton which carry the responsibility of keeping the scientific equipment cool as JWST makes its voyage to L2 [5].
Said equipment includes the NIRCam, NIRSpec, and MIRI— each of which have their own part to play. NIRCam is the primary imager that will detect smaller infrared wavelengths between 0.6 µm and 5 µm in size [10]. Equipped with coronagraphs, it will be able to take photos of exoplanets without being blinded by their stars. Although there are various methods for imaging exoplanets, using coronagraphs helps to image them directly by working similarly to using something to block the sun in order to see the road while driving. This is where NIRSpec takes over: creating spectroscopies of exoplanets to identify their chemical composition [11]. Similar to the coronagraph NIRCam has, NIRSpec has a microshutter system that blocks out irrelevant areas in order to reduce light pollution [11]. However, as stated, NIRCam and NIRSpec work in the near-infrared spectrum, and are therefore more useful in exoplanet research. MIRI is both an imager and a spectrograph, but detects infrared radiation with wavelengths from 5 µm to 28.3 µm in size [12]. These longer waves can travel through clouds of dust that are scattered throughout the cosmos. MIRI will be the instrument for detecting the objects with the highest redshift. However, in order for MIRI to work accurately, its temperature needs to stay below 7 K or it begins to detect its own heat [12].
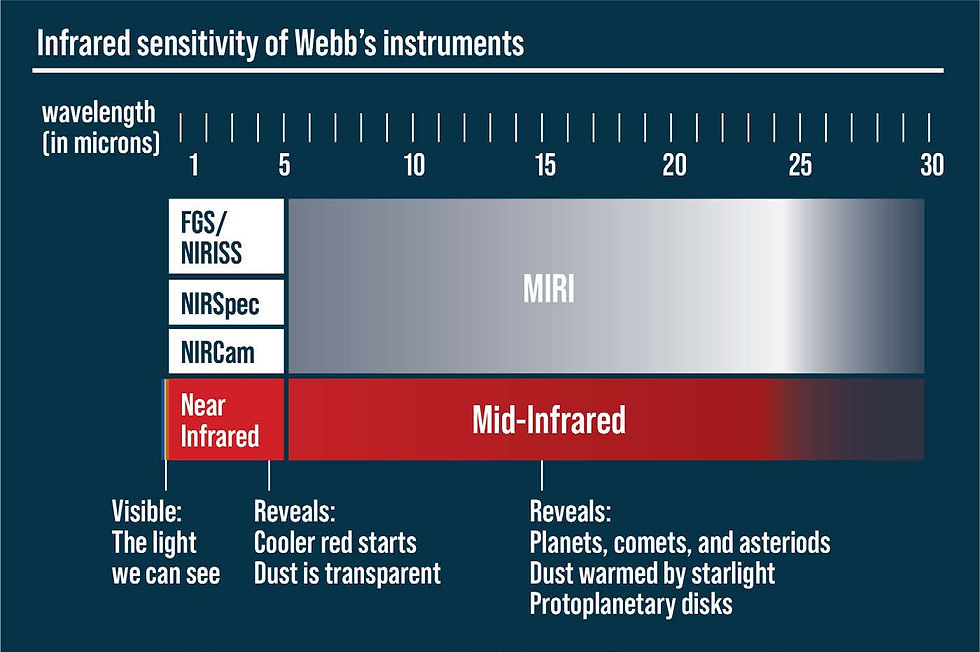
Diagram adapted from NASA's Infrared sensitivity of Webb's instruments
Each piece of the JWST has required multiple decades of designing and testing. With the capability of looking at the universe at a younger age than ever before, the JWST will undeniably change the field of astrophysics. We will be able to peer at the birth of some of the first galaxies, and understand how stars and planets begin to accrue mass. When pointed at nearby stars, such as Trappist, we will be able to see each exoplanet’s atmospheric composition and determine if they are indeed habitable. Possibly, if we are lucky, JWST could identify biosignatures from life on other planets and redefine our existence. Learning about the beginning of the universe and looking for signs of life and habitable planets doesn’t simply fulfill the curiosity we innately have. This knowledge can help us to understand how our own solar system and galaxy was created, how to reveal the mysteries of dark matter, whether life is rare and how it comes into existence, and could even help us with concepts such as how we could terraform other planets such as Mars and Venus. Any findings made by the JWST will certainly build the foundations for future missions or research studies in any range of fields. The JWST is arguably one of the most important inventions of the 21st century with the potential to forever change the way we view the universe and live within it. Launched on Christmas Day of 2021, it is now taking its month-long passage to get to the L2 point [1]. If all goes well, we can expect some incredible new discoveries throughout 2022 and onwards as it looks into the depths of our universe.
References
[1] D. Dobrijevic, “NASA's James Webb Space Telescope: The ultimate guide,” Space.com, 25-Dec-2021. [Online]. Available: https://www.space.com/amp/21925-james-webb-space-telescope-jwst.html.
[2] A. Todd, “Story and Origins of the Universe,” ArcGIS StoryMaps, 06-Apr-2021. [Online]. Available: https://storymaps.arcgis.com/stories/2e16464e7d3549069e13a18c2689ce99.
[3] N. Wolchover, “The Webb Space Telescope Will Rewrite Cosmic History. If It Works.,” Quanta Magazine, 03-Dec-2021. [Online]. Available: https://www.quantamagazine.org/why-nasas-james-webb-space-telescope-matters-so-much-20211203/.
[4] A. May, “James Webb Space Telescope: Origins, Design and Mission Objectives,” LiveScience, 22-Nov-2021. [Online]. Available: https://www.livescience.com/amp/james-webb-space-telescope.
[5] “Comparison: Webb vs Hubble Telescope - Webb/NASA,” NASA. [Online]. Available: https://www.jwst.nasa.gov/content/about/comparisonWebbVsHubble.html.
[6] E. Howell, “Lagrange points: Parking Places in Space,” Space.com, 22-Aug-2017. [Online]. Available: https://www.space.com/amp/30302-lagrange-points.html.
[7] “Cryocooler Webb/NASA,” NASA. [Online]. Available: https://webb.nasa.gov/content/about/innovations/cryocooler.html.
[8] “Ariane 5,” Arianespace, 17-Feb-2021. [Online]. Available: https://www.arianespace.com/vehicle/ariane-5/.
[9] “Mirrors Webb/NASA,” NASA. [Online]. Available: https://webb.nasa.gov/content/observatory/ote/mirrors/index.html.
[10] “Near Infrared Camera (NIRCAM) instrument Webb/NASA,” NASA. [Online]. Available: https://jwst.nasa.gov/content/observatory/instruments/nircam.html.
[11] Perception, “The James Webb Space Telescope Explained In 9 Minutes,” YouTube, 17-Jul-2021. [Online]. Available: https://youtu.be/tnbSIbsF4t4.
[12] B. Kruizinga, H. Visser, J. W. Pel, K. Moddemeijer, and C. Smorenburg, “MIRI spectrometer optical design,” 2004. [Online]. Available: https://adsabs.harvard.edu/full/2004ESASP.554..263K.
Comments